by Rick O'Connor | Apr 11, 2025
Following the textbook I used when I was teaching environmental science there were 6.7 billion humans on the planet in 2011. I remember when we hit 6 billion. There was a lot made of it in the media. Currently (2025) we are at 8 billion. The textbook I used was published in 2011 – which means we have seen a growth of 2 billion people over 14 years – that’s a net gain of 143 million each year – 12 million each month – 400,000 a day – 17,000 an hour – 275 each minute – a net gain of 4 humans every second. Our population growth rate is astounding.
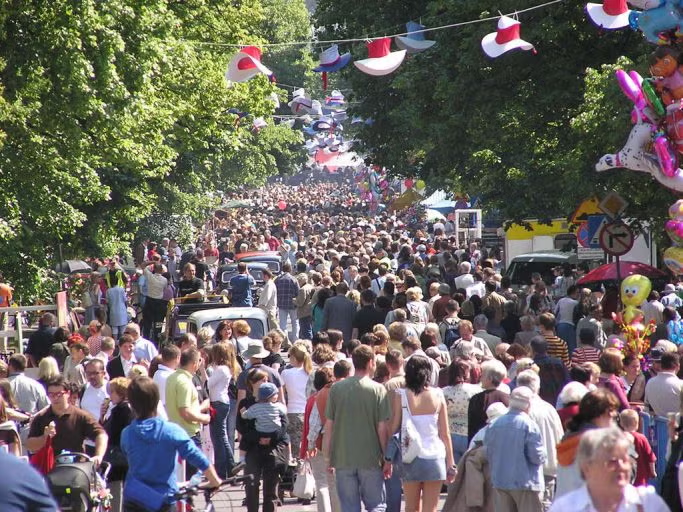
There are nearly 8 billion people on the planet today.
Photo: University of Central Florida
The calculation used to measure population change over time is relatively simple.
Population change = (births + immigration) – (deaths + emigration)
The natural history of creatures on our planet follows the same basic principle. There is a correlation between the number of offspring produced and the amount of parental care provided. The goal of each parent of any species is to have at least one of your offspring reach sexual maturity. Those who give very little parental care – trees, grasses, sea turtles, jellyfish, etc. – will produce large numbers of offspring knowing that over 90% will not make it – many will not make it past the first few days. Those who produce few offspring – songbirds, manatees, and gorillas – provide parental care to assure at least one makes it. Some will provide parental care for a few days, or a few weeks, others will provide it for a few years. BUT even those who show parental care typically produce 2-3 offspring knowing that a couple will not survive.
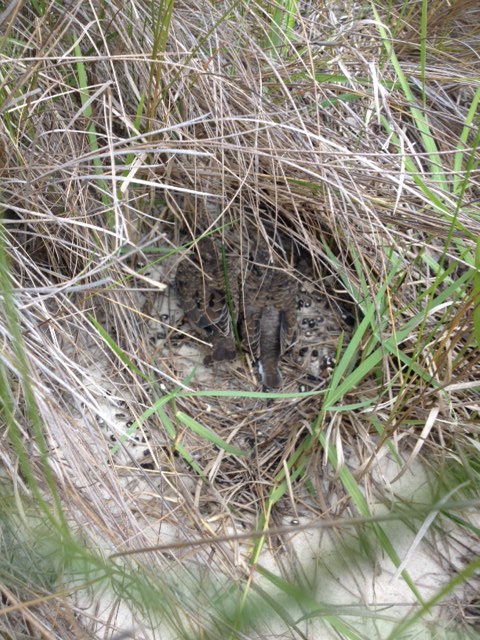
This nest of birds has three chicks. Not all will survive.
Photo: Rick O’Connor
This is a piece of the population control story. It does not benefit any species to have an overpopulation problem. I had to take an oral examination to complete my graduate degree. You are in a chair facing a long table full of professors who are asking you all sorts of questions. One question was “who is a rhinoceros’ greatest competitor?” Being very nervous I was bumbling and fumbling in my brain trying to figure WHO would be their biggest problem… and then – it came to me… another rhinoceros. They want the same space, the same resources, and the same mates as you. Competition begins day one. Over population is a problem for everyone. Other things that effect the number of newborns from reaching sexual maturity are predators and disease. In some species even the parents (typically the father) may be a predator – and will kill their own offspring (polar bears).
Placing humans in this model – we will see that typically there is only one offspring produced. Parents must do all they can to help this offspring reach sexual maturity. For Homo sapiens females reach sexual maturity between the ages of 8 and 13, 9 and 14 for males. In many cultures this is when the parent’s job is over and the child either enters an apprenticeship or gets married. Most cultures do not recognize “adulthood” until the age of 18. So… your job as a parent is to make sure they reach that age.
For most of our history environmental factors played a role in controlling population growth – namely disease. 200+ years ago, many children did not survive childbirth or died early from diseases. But things have changed.
It took from the time humans arrived on the planet to 1927 for us to reach 2 billion humans. It took less than 50 years to add the next 2 billion (1974), and only 25 years at add the next 2 billion (1999). The textbook I used stated we will reach 7 billion by 2012 – we reached it in 2011. They are expecting 9.7 billion by the year 2050.
What happened? What changed to create such a large exponential growth in the human population? The answer lies on both sides of the population change equation – more births are making it to puberty and less people are dying. Modern medicine has done miracles in improving overall human health on both ends – and this is wonderful news, but it does come with a cost. As with any species population – there is only so much space and resources. When the population of a species begins to stress this – many creatures will cease (or slow) reproduction. Another answer we see is dispersal – members of the population will move to new locations and build new populations reducing the stress on the resources. As we mentioned in a previous article – they will spread across the landscape until they reach a barrier that stops them from going further. But in nature the role of predators and disease has not gone away as it has with humans. The current growth rate for humans is 1.1% but has been slowly declining since the 1960s.
This brings up the question of carrying capacity (K) – the maximum number of individuals a population’s space and resources can support. As we mentioned, when K is reached in the natural world species either slow reproduction, disperse, or both. But what IS the carrying capacity for humans on this planet? The textbook I used mentioned there was no consensus on this. Some were saying as low as 2 billion – which we know was not accurate, though some would say some parts of the world are already in a population crisis. Others say it may be as high as 30 billion. A quick search on the internet finds the following…
- The AI response states somewhere between 9-10 billion.
- The Australian Academy of Science states it could be between 500 million and 1 trillion.
- The Population Connection states it is between 500 million and 1 sextillion (21 zeros).
Bottom line – we do not know – it depends on which model you use and how you view/term a functioning population. When I was teaching the class, most models stated somewhere between 10-15 billion.
As we mentioned, when you are reaching carrying capacity – most will either slow/stop reproduction or disperse. In our next article, we will look at how humans might address this problem.
References
Miller, G.T., Spoolman, S.E. 2011. Living in the Environment. Brooks/Cole Cengage Learning. Belmont CA. pp. 674.
Puberty and Precocious Puberty. Eunice Kennedy Shiver Institute of Child Health and Human Development. https://www.nichd.nih.gov/health/topics/puberty.
Population. United Nations. https://www.un.org/en/global-issues/population.
Human Population Growth. Libretexts. https://bio.libretexts.org/Bookshelves/Introductory_and_General_Biology/General_Biology_(Boundless)/45%3A_Population_and_Community_Ecology/45.04%3A_Human_Population_Growth/45.4A%3A_Human_Population_Growth.
How Many People Can the Earth Actually Support? Australian Academy of Science. https://www.science.org.au/curious/earth-environment/how-many-people-can-earth-actually-support.
What is the Carrying Capacity of Earth? The Population Connection. https://populationconnection.org/blog/carrying-capacity-earth/.
by Rick O'Connor | Mar 28, 2025
Evidence suggests that Homo sapiens’ initial population originated in east Africa – though some think we may have originated in southern Africa, and others from northwest Africa. It is believed this occurred about 300,000 years ago. The first humans were hunter gathers and fed primarily on small prey and plants – we are omnivorous. With the development of stone tools early humans could feed on larger prey and take a different position in the food chain.
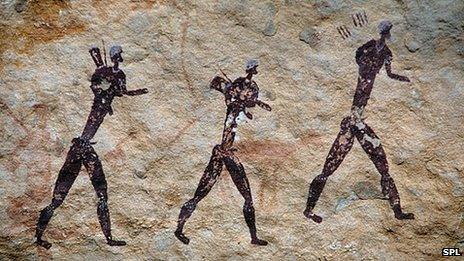
Evidence suggest that humans originated in Africa and began their dispersal across the planet from there.
Image: BBC.
Populations of all creatures increase and decrease due to the number of births, deaths, immigration, and emigration within their populations (we will focus more on the human population in the next article). As populations grow, competition for needed resources increases. One response to this competition is dispersal – the movement of members of a population to a new location where resources can be found. This is different than migration in that in dispersal the members do not return – they have moved away.
Evidence suggests that humans began dispersing from Africa very early. It was thought at first humans did move into southern Africa, but there was a mass movement north into the Middle East. From here humans began to move east along southern Asia to India and beyond to southeast Asia. There is evidence of “island hopping” as they made their way across Indonesia and eventually to Australia. Later groups from southern Asia dispersed north into northern Asia. From here there were two movements – one into Europe and another across an exposed landmass connecting Asia to North America – remember the Earth continues to go through slow change over time. At one time there was a land bridge that connected the two continents and allowed humans, and other species, to cross. Humans began to spread across North America and – with the emergence of a land bridge in Central America – reached South America. There are those who also believe – based on language and culture – some Polynesian humans reached South America by boat. To test this idea an expedition sailed from Polynesia to South America on a balsa wood raft called Kon Tiki in the 1940s. This same team attempted to sail from Africa to South America to show this could have been a dispersal route as well using boat materials from that time – but that expedition failed. However they reached South America, they did – and humans had dispersed to cover all landmasses except Antarctica. This still holds true – there are no native populations of humans on the Antarctic continent – only visitors.
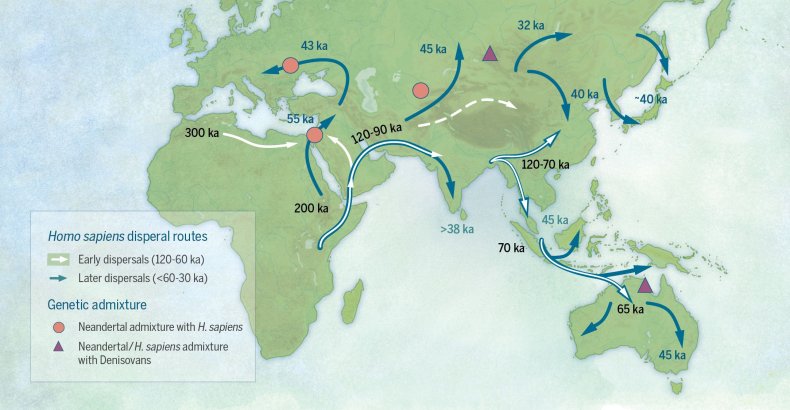
This shows possible dispersal routes of humans out of Africa.
Image: Newsweek.
Within each region of the planet where humans have inhabited, we find physical and cultural differences. The physical difference – which we call races today – were adaptations to the environment where they lived. There languages, tools and building practices, songs and instruments, and religions were all born from the area where they lived. Many of these populations were isolated from each other – and so their cultures became quite different. As these populations grew and expanded, they would come in contact with each other. Many times, these different cultures, or tribes, would fight for resources and space. As our imaginations and technologies grew, some cultures were able to cover more territory and conflicts increased.
Today there are about 8 billion humans on the planet. Some areas are more densely populated than others. Based on the website Worldometer – we are gaining a new human almost every second.
Over history, most species have had a slower population growth – if growing at all. Numbers are kept under control by predators, disease, and environmental conditions that impede, or restrict, reproduction. But not with humans. In the next article we will look closer at the cause of the human population explosion.
References
Pavid, K. 2018. Rethinking Our Human Origins in Africa. Science News. Natural History Museum. https://www.nhm.ac.uk/discover/news/2018/july/the-way-we-think-about-the-first-modern-humans-in-africa.html.
Pobinar, B. 2013. Evidence for Meat-eating by Early Humans. Nature Education Knowledge. (46)1. https://www.nature.com/scitable/knowledge/library/evidence-for-meat-eating-by-early-humans-103874273/#:~:text=Tooth%20morphology%20and%20dental%20microwear,Ungar%202000%3B%20Luca%20et%20al..
Dorey, F., Blaxland, B. 2020. The First Migrants Out of Africa. Australian Museum. https://australian.museum/learn/science/human-evolution/the-first-migrations-out-of-africa/.
Kon Tiki Expedition. Wikipedia. https://en.wikipedia.org/wiki/Kon-Tiki_expedition.
Worldometer. https://www.worldometers.info/world-population/.
by Rick O'Connor | Mar 7, 2025
The principles of life on land are not that different from life in the sea. It is believed that life began in the sea but, the creation of continents and islands, new habitat was available for colonization. However, the creatures who would colonize land would have to have different characteristics than those in the sea.
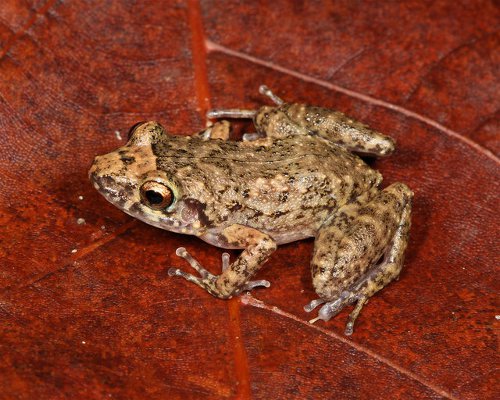
Frogs are land animals that use water environments as well.
Photo: U.S. Geological Survey
A limiting factor for the producers in the sea was light. Sunlight is the energy that fuels the process of photosynthesis. Thus, the producers had to either live in shallow water or be able to stay near the surface of the sea in order to function. For MOST locations on land, sunlight is not the issue. In fact, in some locations, too much sunlight can be an issue. You need sunlight, water, and carbon dioxide to photosynthesize – and on land, water would be the hardest to obtain. Many land creatures actually still live in water part time. There are rivers, lakes, and streams crossing over the landscape that can provide suitable habitat for both producers and consumers. However, most of the landscape is not water and photosynthesizing plants – like trees, grasses, and shrubs – developed root systems to absorb water from the ground.
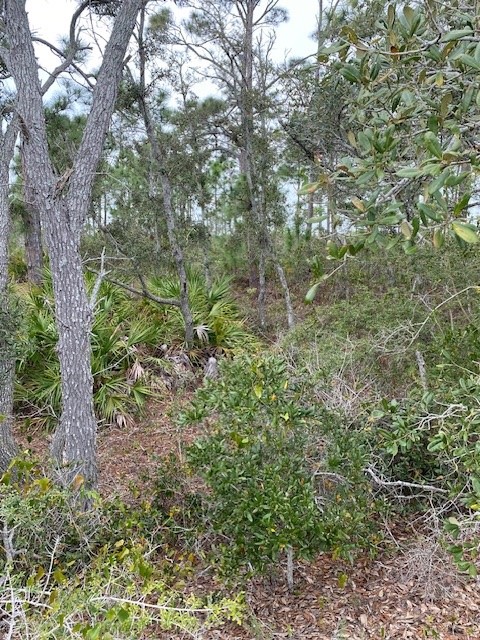
Forest and trees are plants that have adapted to obtaining water from the ground.
Photo: Rick O’Connor
Another player in this game are the swirling ocean currents. As they rotate past the polar regions of the world, they become colder. As the cold currents pass land, they lower the humidity and produce less rainfall. Drier conditions meant plants had to adapt to both the issue of over evaporation and sufficient groundwater to support them. Desert plants have done just that. Often along mountain ranges the mountainside closest to the ocean, or warm currents will produce the greatest amount of precipitation. While the opposite will be drier and more desert like. The producers who live on each side of the mountain will be different due to this. Landscapes near warm ocean currents are humid and rainfall is high. Here the landscape is lush and green with some of the highest plant diversity found on the planet.
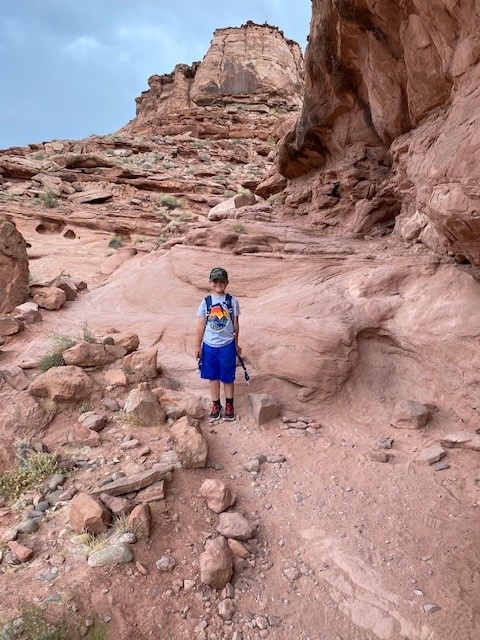
Deserts are found in locations where rainfall and humidity are low.
Photo: Rick O’Connor
Land dwelling consumers follow suit. They must first be able to deal with the amount of sunlight, temperature fluctuations, and humidity as the planets do. Then the primary consumers – those that feed on the producers – must be able to feed on the plants that have adapted to that area.
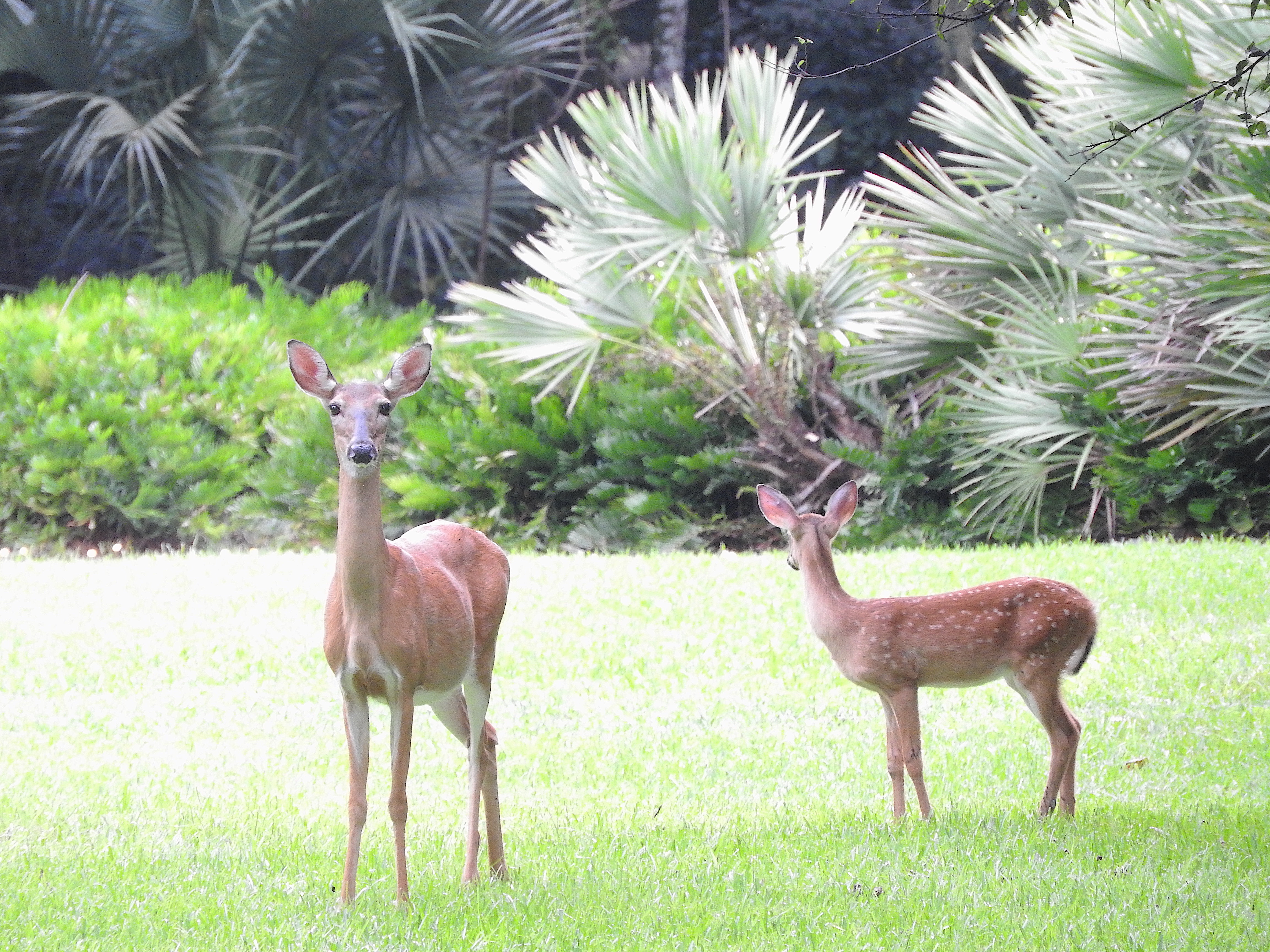
Deer are land animals that have adapted to several climates and feeding on land plants.
Photo: Carolina Baruzzi.
These climate conditions have formed what biologists call biomes – ecologically unique ecosystems. Examples of biomes are deserts, grasslands, alpine forests, hardwood forests, swamps, marshes, and more. Each possesses their unique plant and animal communities. And – like the systems found in the sea – they are constantly changing. Life on land has to deal with the seasons of the year more than those in the oceans do. Prey evolve to defend themselves from predators. Predators evolve to overcome these new defenses. Some species are very successful, others have gone extinct. Though large-scale climate changes – such as ice ages and warming periods – impact life in the sea, they tend to be more impactful for life on land.
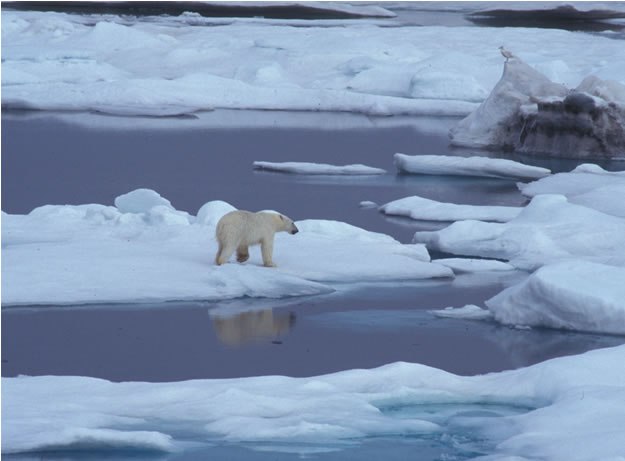
Polar bears have adapted to harsh conditions on land.
Photo: NOAA
Biogeography is the study of how creatures disperse across the landscape (or ocean), meet barriers, and define their biological range. Some creatures have large areas where they are found – such as seagulls, squirrels, and raccoons. These are called cosmopolitan species. Others have very small ranges – such as the marine iguana, Komodo dragon, and kangaroos – and are referred to as endemic species. There are many plants and animals that dispersed across North America and can be found on both coasts. Others are only found in one small area of the country. In our next article we will introduce one species that has dispersed across the entire planet – humans.
by Rick O'Connor | Feb 21, 2025
They say life began in the oceans. We know that the lithosphere is cracked, adding new land, subducting land over time. But much of the lithosphere is covered with water – and here life began. Initially it had to begin on either rock or sand. The sand would have been produced by the weathering and erosion of rock. Obviously, this would all have had to occur over a long period of time. But the first forms of life would have to be able to find food and nutrition from a barren seafloor with little to offer. This would take a special community of creatures – ones we call the pioneer community.
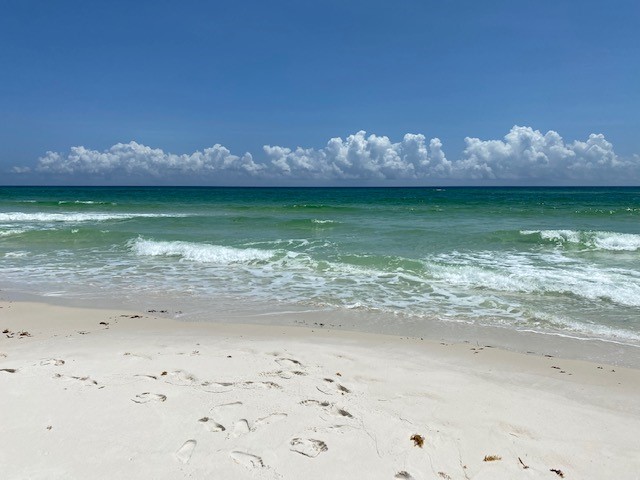
It is believed that life began in the ocean.
Phot: Rick O’Connor
Key members of these communities would have been the producers’ ones who produce food. What we know now is that producers absorb carbon dioxide and water and – using the sun as a source of energy – convert this into carbohydrates and oxygen. We have since learned that there are ancient forms of bacteria that can do this with hydrogen sulfide and other compounds. However, it started – it began. One problem with this model is that much of the world’s oceans are too deep for sunlight to reach. Thus, living organisms would need to be close to shore. Today we know two things. One, the ocean’s surface is covered with microscopic plant-like creatures (phytoplankton) who can float and reach the sunlight. Two, some of the ancient chemosynthetic bacteria (those that do not need the sun and can use other compounds to produce carbohydrates) live on the ocean floor.
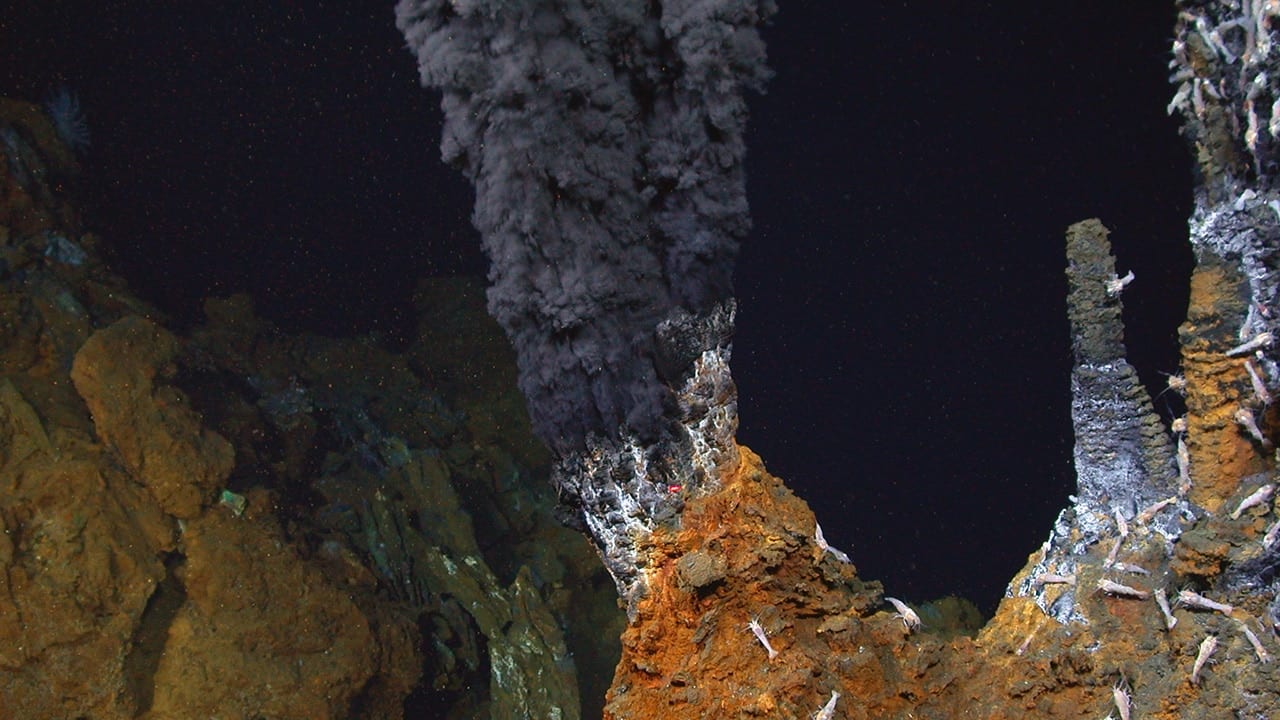
The black smokers – hydrothermal vents – found on the ocean floor.
Photo: Woodshole Oceanographic Institute.
Producers are followed by consumers, creatures who cannot make their own food and must feed on either the producers or on other consumers. There are plankton feeding animals – oysters, sponges, corals, and zooplankton. There are larger creatures that feed on larger plankton – manta rays, menhaden, and whales. There are consumers who feed on the first order of consumers – stingrays, parrotfish, and pinfish. And there are the top predators – orcas, sharks, and tuna. The ocean is a giant food web of creatures feeding on creatures. All creatures evolve defenses to avoid predation. Predators evolve answers to these defenses. Some species survive for long periods of time like the horseshoe crabs and nautilus. Others cannot compete and go extinct.
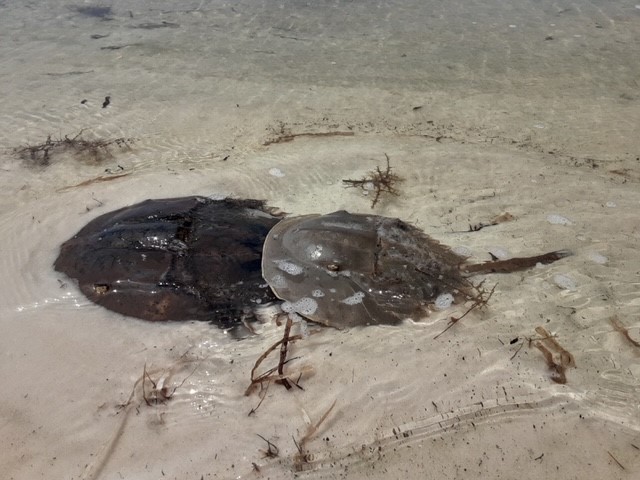
Horseshoe crabs are one of the ancient creatures from our seas. Photo: Bob Pitts
As we mentioned in Part 1 of this series – the hydrosphere is in motion. Different temperatures, pressure, and the rotation of the planet move water all over. These currents bring food and nutrients, remove waste, and help disperse species across the seas. Life spreads to other locations. Some conditions are good – and life thrives. Others not so much – and only specialists can make it. The biodiversity of our oceans is an amazing. Coral reefs, mangrove forests, and seagrass beds are home to thousands of species all interacting with each other in some way. The polar regions are harsh – but many species have evolved to live here, and the diversity is surprising higher than most think. The bottom of the sea is basically unknown. It has been said we know more about the surface of the moon than we do at the bottom of our ocean. But we know there is a whole world going on down there. We believe the basic principles of life function there as they do at the surface – but maybe not!
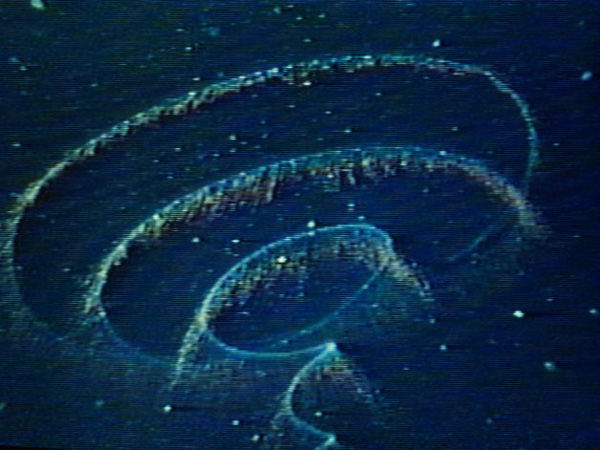
The magical lights of the deep sea.
Photo: NOAA
Fossil records suggest life here began almost one billion years ago. The fossils they find are of creatures similar and different from those inhabiting our ocean currently. As we stated that the physical planet is under constant change – life is as well. It is a system that has been working well for a very long time. Over the last few centuries humans have studied the physical and living oceans to better understand how these systems function. They have been functioning well for a very long time. And though life began at sea – there was dry land to exploit – for those who could make the trip. That will be next.
by Rick O'Connor | Feb 14, 2025
To better understand the basics of how the planet works we will use the analogy of a chicken egg. The yolk would represent the core – solid and in the middle of the planet. The mantle would be like the egg white that surrounds the yolk. It takes up most of the space, is fluid, and slowly rises and falls as it is heated by the core. The eggshell would represent the crust of the earth. Solid, and very thin. However, the crust of our planet is not smooth. It is covered with mountains and valleys, some of it above sea level but most (70%) would be below the oceans. And above the eggshell would be a thin layer of gas we call the atmosphere.
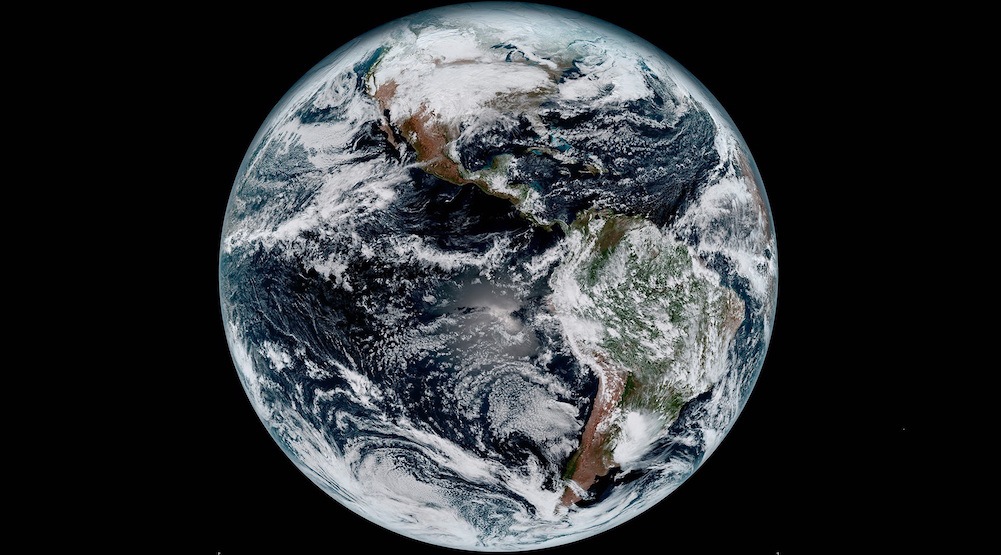
Our fragile Earth
Photo: NOAA
What we know of the core of the planet has come from seismic waves we have generated. As sound waves pass through different materials, the waves bend and reflect differently. The patterns of the refraction and reflection suggest that the core is made of iron and nickel. The temperatures here are extreme – up to 9000°F – but the intense pressure generated by gravity keeps the inner core a solid – like our egg yolk.
The mantle is actually solid rock but moves VERY slowly like a thick liquid – similar to glaciers. Like the core – the mantle is very hot but the pressure there keeps in a more solid fluid state. However, as this rock layer comes closer to the crust, the temperature is high enough, and the pressure low enough, that it becomes more fluid like – and we call it magma. This upper portion of the mantle is known by geologists as the asthenosphere.
The crust is made of solid igneous rock that is basically cooled magma. Granite is the rock that makes up the continental land masses and the denser basalt is found on the ocean floors – basalt “sinks” deeper into the more fluid mantle. Geologists call the crust the lithosphere.
The ocean is made of 97% water and 3% salt. This saltwater makes up 90% of the water on the planet. Freshwater can be found on land and in the ice of the polar regions. They refer to all of the water on the planet as the hydrosphere.
The air above the planet is a thin layer of gases made primarily of nitrogen, but also includes oxygen, carbon dioxide and other trace gases. It is called the atmosphere.
If you were to look down on the planet from the north pole it would rotate counterclockwise – from the south pole it would be clockwise. This rotation causes the hydrosphere and atmosphere to “slush around”. Add temperature and pressure change as a cause for this rotation we generate the winds and currents of our planet.
Over the last century scientists have discovered that the crust is cracked, and each section of eggshell is called a plate. These cracks provide places where molten magma can exit into the environment in the form of volcanos. These can be found on both land and at the bottom of the sea. The molten rock cools when it meets the hydrosphere and atmosphere and a cocktail of gases, liquids, and solids are produced. These gases contributed to the formation of the atmosphere that was held close to the planet by gravity.
About one billion years ago some of these molecules formed cell like structures that could take in gases, liquids, and solids and release waste products. This taking in/converting/releasing out of new materials changed the chemistry of the land, sea, and air. Oxygen increased in the atmosphere and the planet cooled to a point where these cell-like structures could live, grow, and reproduce. They produced their own energy to maintain their own metabolism and eventually some cells developed that could not make their own energy and needed to consume the cells that did – predator/prey relationships were developed.
As the planet aged and changed, the living creatures aged and changed. Many creatures could not survive the new changes and went extinct. With the new changes, new creatures would appear. Those had to deal with the conditions of either land, sea, or air and with the other creatures that lived nearby. Interactions between these creatures would change the environment and the process of change in life followed suit.
This is how our planet has functioned since its beginning. The planet changes, the living organisms change, these changes cause more changes, and the planet evolves over time. Today, there are millions of species on the planet and millions more have gone extinct. In the next article we will look at how these systems function in the hydrosphere.
by Rick O'Connor | Jan 31, 2025
Each year I survey the public to determine what their needs are – what they are most concerned about – and where Extension might help. One of the primary functions of Extension is to provide local clientele with solutions to their needs – our mantra at UF IFAS Extension has been “Solutions for Your Life”. Most years the primary concern for Sea Grant locally has been water quality. Habitat restoration and invasive species also score high. These are all important issues. We want to maintain a high quality of life and addressing these concerns will help do that. And as important as these are, and they are, if someone were to ask me about my highest concern, it would be climate change. The issues above can be connected directly, or indirectly, to climate change.
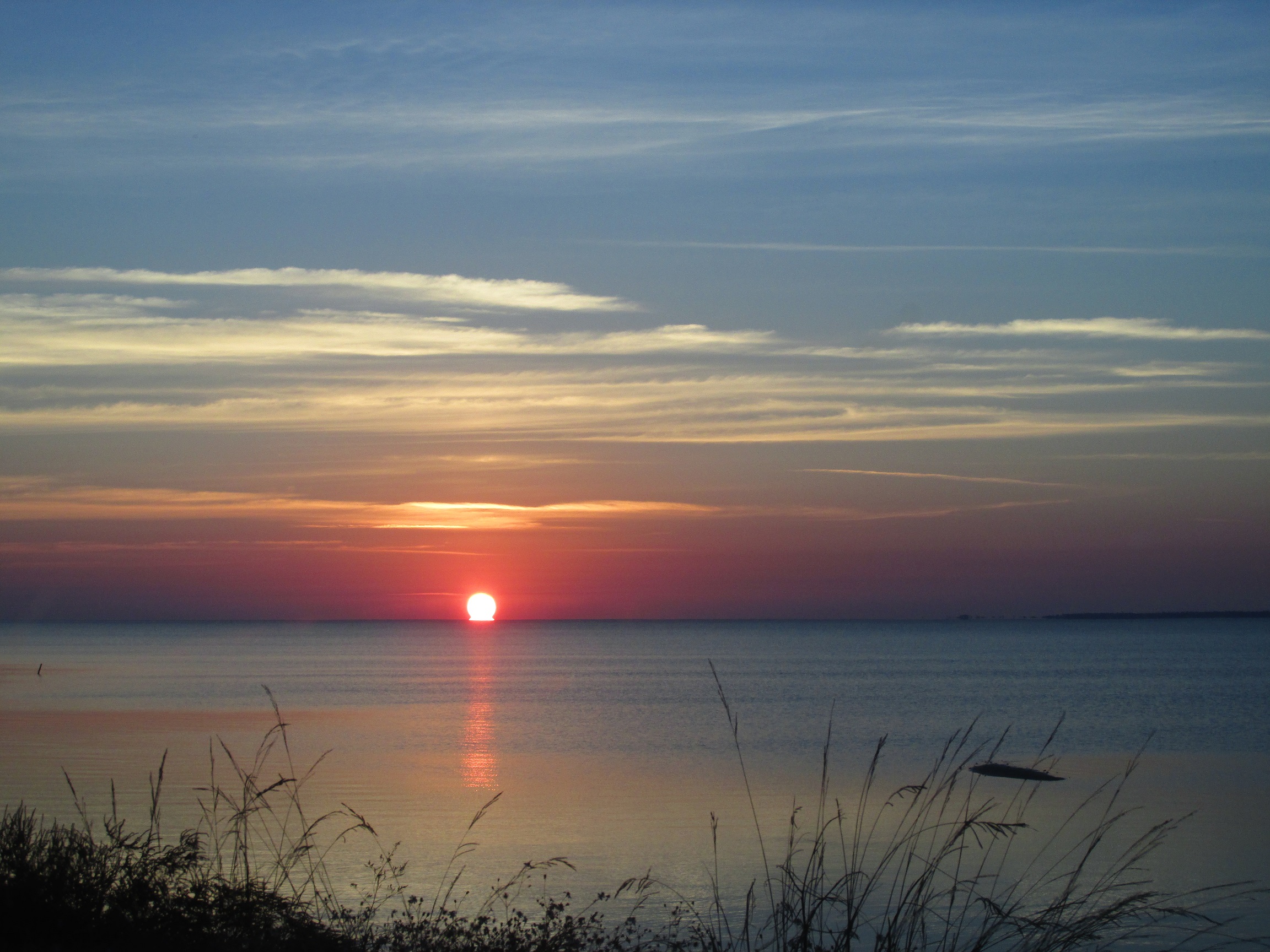
Sunrise over Apalachicola Bay in Northwest Florida
Scientists have predicted, and witnessed, the negative impacts of climate change for several decades now. Nonscientists have begun to notice as well. Increased number of hurricanes, increased intensity of some of these hurricanes, excessive and extended heat waves in the summer, drought conditions in some areas of the country, flooding issues in others, and wildfires out west. These things have all happened before but in recent decades they are becoming more common.
In 2024 I wrote four articles about our changing climate in response to the devasting hurricane season we had. The reason was my concern about climate change and that we all need to pay more attention to the issue. I wrote a total of 41 articles over the course of the year – including these four on climate. The articles on climate were the least read articles of the group. There could be a couple of reasons for this. It could be that most do not consider it a high priority concern – and I understand that. Again, water quality and habitat loss are real concerns and climate maybe less so for many people. It could be that people understand the concern about climate but what can you do? It is too big of an issue for me to deal with and so, I will focus on what I can do something about. It may be because the topic is so negative – and I get this also. I taught environmental science at Pensacola State College for 15 years. Despite there being many positive lessons in the course, many students were so depressed by the final exam they would add notes such as “I will never have children” and others similar to this. Discussing the state of our environment can be a “downer”, and they just do not want to deal with it. And it could be that they deny climate change is even happening. There are many who do feel this way. In their minds, there is no issue, nothing to discuss, nothing to read about – move on.
I posted my four articles and let it ride (links to them are posted below). However, with the tragedy of the fires in Los Angeles recently, I felt it needed to be brought back up. I watched a program about the fires and a science writer, who recently published a book about climate, made a statement to the effect that “nature is letting us know that something serious is going on… nature is letting man know that we are in this together and we need to make some changes”. I agree. Though the topics of water quality and habitat loss are concerning – and Extension will provide articles and programs during the year to help the community solve those problems – I also believe that the community needs to pay more attention to climate change and enact behavior changes to solve this very serious problem.
I have decided to post articles throughout 2025 mimicking the environmental science course I used to teach. I am obviously going to shorten the subjects – and may write more in-depth articles about some of them down the road – but the outline will follow that of the course. We will begin with how the planet works. This will be followed by the introduction of humans and how we have spread across the planet. There will be articles about how we have impacted our natural resources and the climate. And we will end with some suggested methods for solving some of the problems. I am not expecting a lot of people to follow this – but I hope you will. And I hope some positive change will come from it. Let’s begin…
Climate articles from 2024
Another Look at Climate Change – Part 1 Introduction
https://blogs.ifas.ufl.edu/escambiaco/2024/06/20/another-look-at-climate-change-part-1-introduction/.
Another Look at Climate Change – Part 2 How Might the Earth’s Temperature and Climate Change in the Future?
https://blogs.ifas.ufl.edu/escambiaco/2024/06/25/another-look-at-climate-change-part-2-how-might-the-earths-temperature-and-climate-change-in-the-future/.
Another Look at Climate Change – Part 3 What are Some Possible Effects of a Warmer Atmosphere?
https://blogs.ifas.ufl.edu/escambiaco/2024/07/03/another-look-at-climate-change-part-3-what-are-some-possible-effects-of-a-warmer-atmosphere/.
Another Look at Climate Change – Part 4 What Can Be Done?
https://blogs.ifas.ufl.edu/escambiaco/2024/07/08/another-look-at-climate-change-part-4-what-can-be-done/.